Biggest, Youngest Black Hole Ever Shocks Astronomers
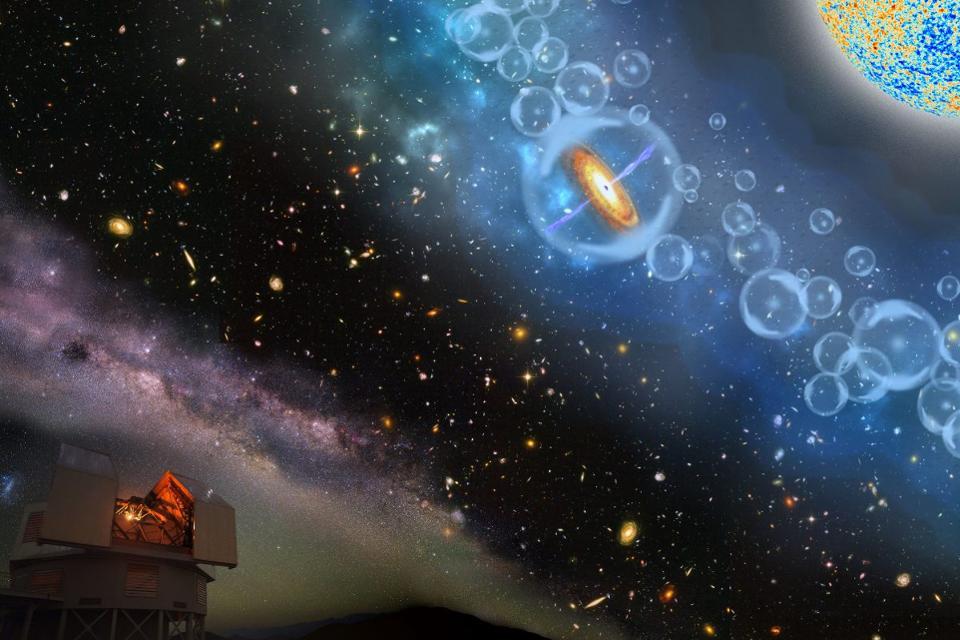
The farther away we look, the closer in time we travel back towards the Big Bang. The latest … [+]
Robin Dienel/Carnegie Institution for Science
In every scientific field, we’re always on the lookout for whatever new discovery might reveal what’s currently beyond the known frontiers. Searches for smaller, more fundamental particles, temperatures ever-closer to absolute zero, or distant objects at the recesses of the Universe help drive our progress forward. Where our observations or measurements give us a result that we weren’t theoretically anticipating, that’s the most exciting moment for a scientist, as it’s often an omen that we’re about to learn something wholly novel about the Universe we inhabit.
At the 237th meeting of the American Astronomical Society, scientist Feige Wang announced the discovery of a new quasar: an active, ultra-bright, supermassive black hole found at the centers of distant galaxies. This is the most distant quasar, and hence the most distant black hole, ever found. Its light comes to us from when the Universe was just 670 million years old, or ~5% of its current age, and yet it already grew to a mass that’s a whopping 1.6 billion times as massive as our Sun. It’s a mystery how a black hole this big can exist this early on, presenting both a crisis and a unique opportunity for astronomers and astrophysicists.
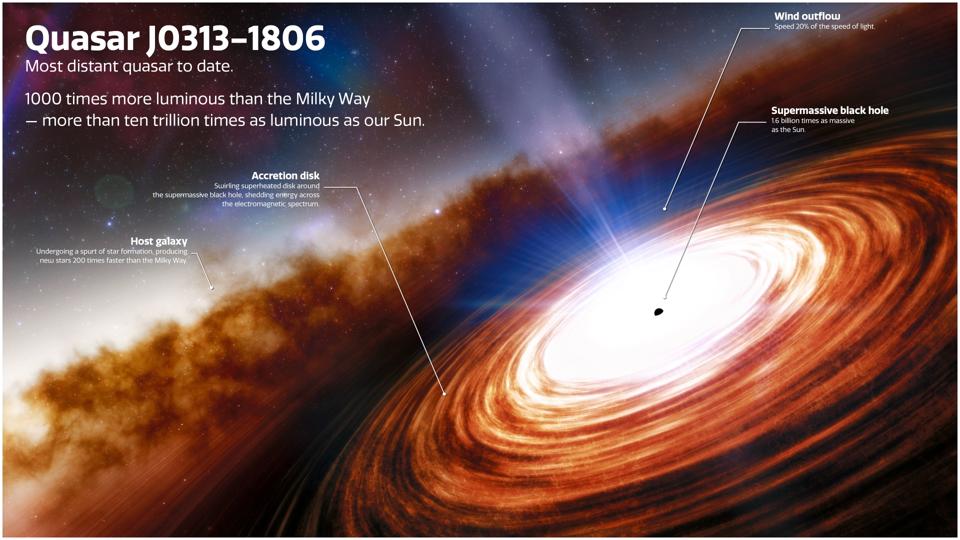
An artist’s impression of quasar J0313-1806 showing the supermassive black hole and the extremely … [+]
NOIRLab/NSF/AURA/J. da Silva
If we were to rewind the clock all the way to the very early Universe, shortly after the Big Bang, we know that we’re looking back to a time before there were galaxies, stars, or black holes of any type. Although there might be a trickle of stars forming between 50 and 100 million years after the Big Bang, the first major burst of star formation isn’t expected to occur until closer to 200 million years after the Big Bang. The first stars are expected to be massive, with many stars reaching hundreds or even a thousand or more solar masses.
When those first stars die, which occurs after only a few million years, many of them will collapse into black holes, either through a core-collapse supernova or through a different direct-collapse process. The early star clusters that house these young black holes occur in the regions of space that started off with significantly more matter than average: the most overdense regions of all. Over time, they’ll attract more and more matter into them, leading to the formation and growth of galaxies, bursts of new stars, and enabling those initial black holes to grow.
MORE FOR YOU
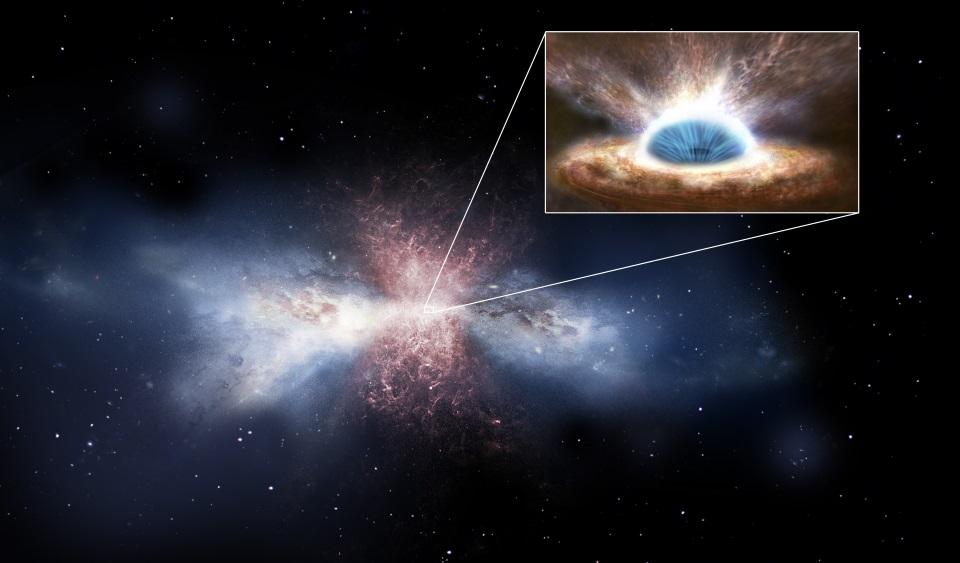
This artist’s rendering shows a galaxy being cleared of interstellar gas, the building blocks of new … [+]
ESA/ATG Medialab
But how fast can they grow? The largest black holes we find in the Universe today are a few tens of billions of solar masses, indicating that they devoured a tremendous amount of matter and/or merged with an enormous number of other black holes in order to achieve those masses some 13.8 billion years after the Big Bang. When we look back into the early Universe, however, the galaxies we see are smaller, lower in mass, and possess younger populations of stars than their modern day counterparts.
Put simply, the Universe needs enormous amounts of cosmic time to pass for structures to grow and evolve. There’s a limit to how quickly stars can form, since star formation heats up the surrounding matter, while star formation itself requires cool gas. The most massive black holes that form will sink to the center through gravitational interactions with lighter masses, where they’ll merge together to form the first supermassive black holes. And when matter falls into those black holes, it gets heated and accelerated, producing energetic jets that further help to suppress future star formation.
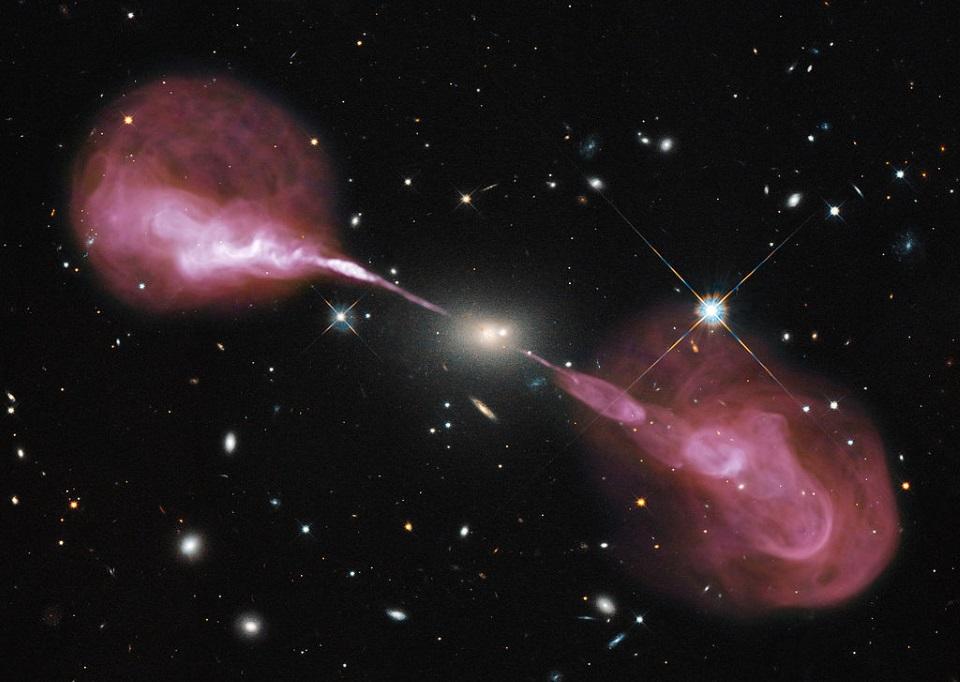
While distant host galaxies for quasars and active galactic nuclei can often be imaged in … [+]
NASA, ESA, S. Baum and C. O’Dea (RIT), R. Perley and W. Cotton (NRAO/AUI/NSF), and the Hubble Heritage Team (STScI/AURA)
When we put all of this together, quantitatively, we fully expect that there will be a maximum limit — at least, if our standard theories and our applications of them are correct — to how massive a black hole could possibly get at early cosmic times. About two years ago, scientists discovered a then-record black hole from just 690 million years after the Big Bang that had a mass of 800 million (0.8 billion) solar masses, which itself was a challenge to explain.
The seed black holes would have had to form from the very first stars, and then grow at what astronomers presently think is the maximum rate possible, the Eddington limit, for the entirety of its existence in order to reach this mass value so quickly. According to an analysis done in that very paper, they expected only about ~20 such black holes of that magnitude in the entire Universe by that time. The odds that we would have found even one, given the narrow portion of the sky that had been surveyed so deeply, were unfavorable.
Now, a couple of years later, we have a black hole from another 20 million years earlier, and it’s fully twice as massive as the previously most distant quasar.
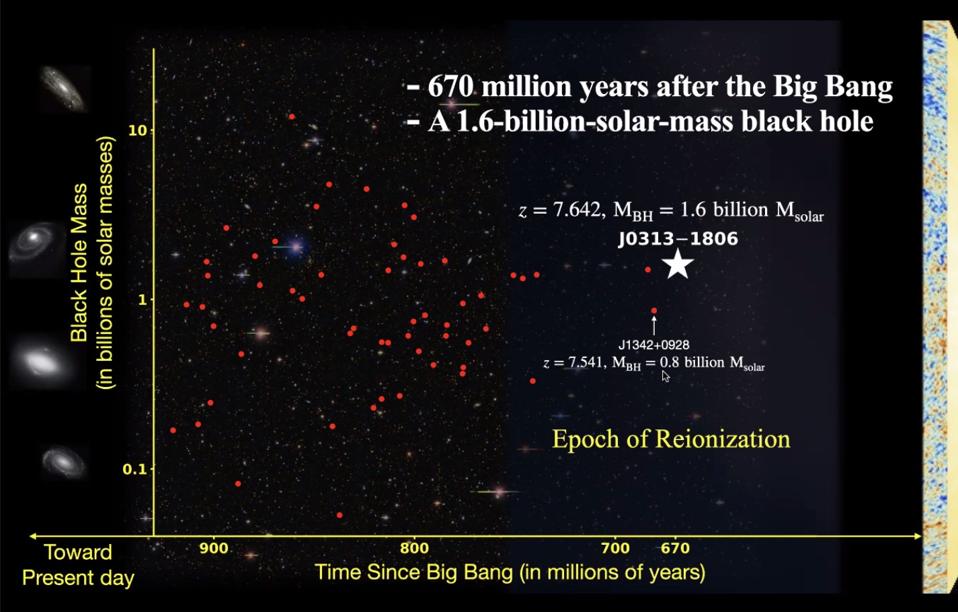
The new record holder for the earliest black hole as compared with the previous record holder and a … [+]
Feige Wang, presented at AAS237
For an object like this, we absolutely have to ensure that we aren’t fooling ourselves. It isn’t enough to gather the light from a distant object and determine that it has no ultraviolet or visible light emission, but rather is so reddened that it falls into the infrared; there are a number of foreground effects that could give you the type of signal we observed. What you have to do, if you want to be certain, is to break the light up into its individual wavelengths, determining its spectra and identifying a variety of features.
When the researchers did this for this particular new quasar — J0313-1806 — was to take a spectrum of this object, and they identified four key features when they did so.
- An emission of the Lyman-α line, coming from energetic hydrogen, which occurs at a specific wavelength: 121.5 nanometers.
- A spectral feature corresponding to triply-ionized carbon, which is present in energetic environments where stars have already lived-and-died.
- Another feature pointing to the presence of singly-ionized magnesium, indicating the prior presence of massive stars that have already died.
- And two asymmetric “dips” that correspond to absorption features at two different wavelengths: one a little longer and one a little shorter than the other.
The fact that we observed all of these features in the infrared portion of the spectrum tells us how distant and ancient this light truly is.
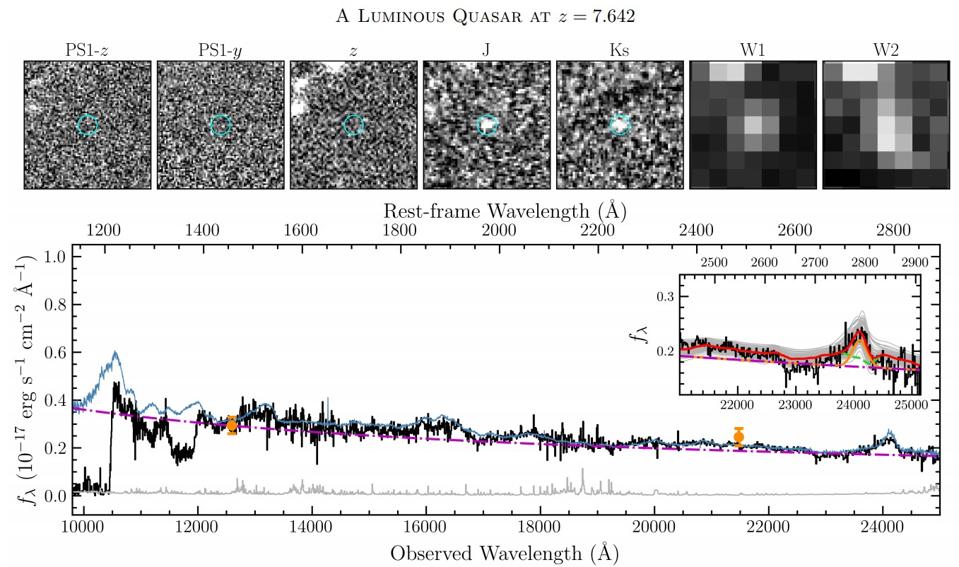
The spectroscopic breakdown of the light from quasar J0313−1806 shows Lyman-α emission, two … [+]
Feige Wang et al. (2021), arXiv:2101.03179
Those two dips are particularly interesting, though. There are a bunch of questions we can ask about those absorption features, and answering those questions leads to a fascinating conclusion.
What absorbs light at a particular wavelength? Neutral gas, whose atoms get excited by light of a particular energy, but then re-radiate in a random direction, preventing that light from reaching our eyes.
Why would there be two different absorption features? If the gas is moving in two directions — one towards us and one away from us — you’ll get two separate features peaking at two different wavelengths.
Why would the absorption features be broad instead of narrow? Because the gas either moves with varied velocities (over a range) or is heated to a significantly high temperature.
So what could be causing this? What’s the one feature common to quasars and active galaxies? Two fast-moving, relativistic jets of matter moving in opposite directions. This is a quasar, and these absorption features are what we know as quasar outflows.
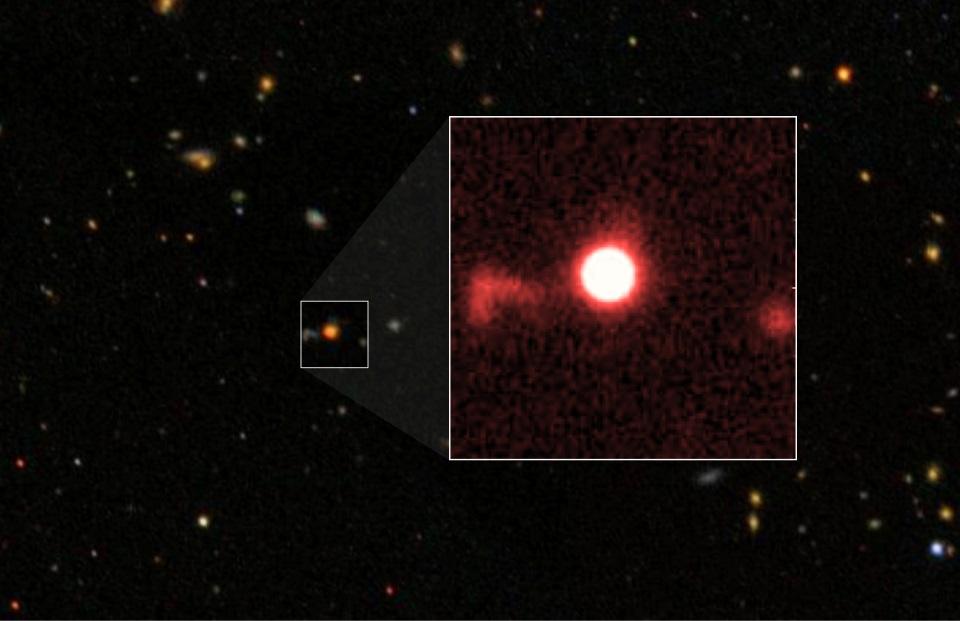
The active galaxy IRAS F11119+3257 shows, when viewed up close, outflows that may be consistent with … [+]
NASA’s Goddard Space Flight Center/SDSS/S. Veilleux
This quasar was initially found by the Magellan telescope, and then was spectroscopically confirmed by the Gemini telescope, which determined its redshift, distance, and a host of other parameters. This quasar is:
- as luminous as 36 trillion Suns over all wavelengths of light,
- just one-tenth the radius of the Milky Way, only perhaps 5,000 light-years from the center to the edge,
- undergoing a large and continuous amount of star-formation, averaging ~200 new solar masses worth of stars per year,
- extremely dusty, with about 70 million solar masses worth of dust,
- with two jets moving quickly in opposite directions: one moving at ~14% the speed of light and the other moving at ~18% the speed of light,
- containing a supermassive black hole that’s grown to 1.6 billion solar masses,
- whose light has been journeying to us for the past 13.1 billion years,
- and that’s presently located, accounting for the expansion of the Universe, 29.4 billion light-years away.
What’s remarkable about this is the fact that, even if we assume this black hole grew at the maximum rate we believe is possible, and did so from the very first stars/black holes, it requires a “seed” black hole of about 10,000 solar masses, which is perhaps a factor of 10 more massive than the most massive black holes we expect to exist at that time.
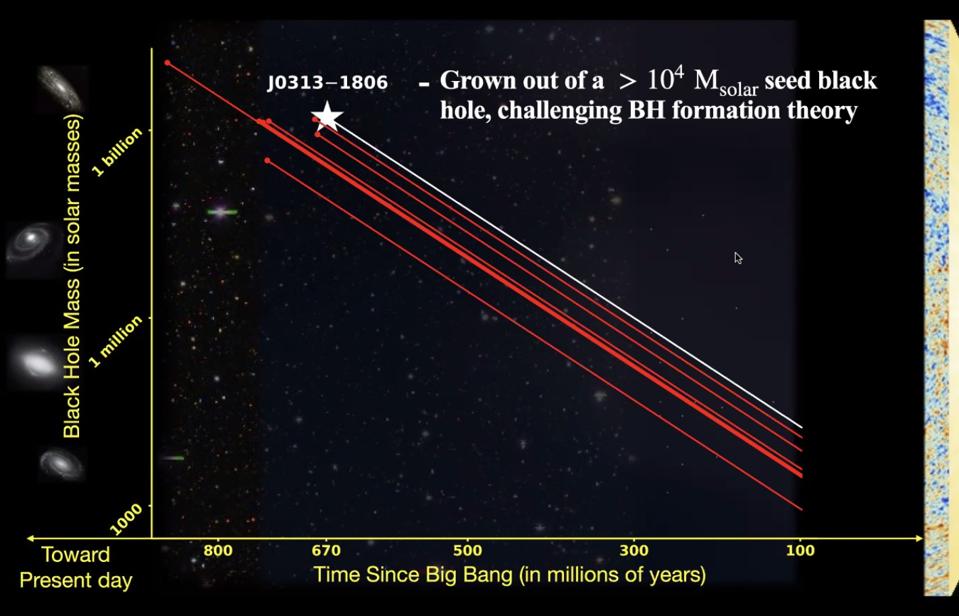
If you begin with an initial, seed black hole when the Universe was only 100 million years old, … [+]
Feige Wang, from AAS237
What’s also surprising about this quasar is that its high level of activity — the energetic, relativistic jets, its high luminosity, and the enormous energy output associated with it — is coupled with very high ongoing levels of star formation. This, as far as we understand it, shouldn’t be possible for long.
What always happens in systems like this is something called “quenching,” which is where energy injection from one process prevents another process from continuing. To have star formation, for example, you need lots of cold gas gravitationally collapsing to form new stars. If you were to inject a lot of energy into that gas, it would heat up and wouldn’t be able to collapse. Quasars, and particularly the jets and other emissions from that quasar, should be doing exactly that.
In other words, quasar outflows should be quenching star formation in this object. And yet, it appears to still be growing and forming new stars at a spectacularly fast rate: 200 solar masses worth per year.
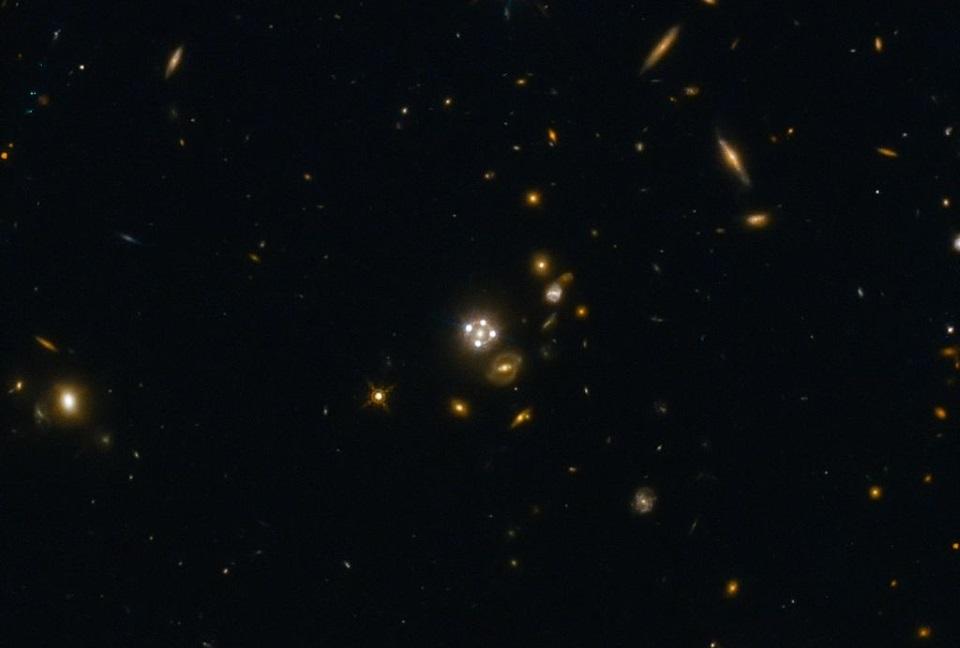
HE0435-1223, located in the center of this wide-field image, is among the five best lensed quasars … [+]
ESA/Hubble, NASA, Suyu et al.
Fortunately, we have the technological capacity coming to learn much, much more about what’s going on in this distant object that appears to defy conventional explanation. We haven’t been able, even with the best ground-based infrared telescopes that we have, to image either the host galaxy itself or the quasar outflows directly.
The Atacama Large Millimetre/submillimetre Array (ALMA) is an extremely large and powerful array of radio telescopes, and it specializes in imaging the gas and dust present in externally heated environments. If we want to measure the outflows from this quasar system, ALMA — as soon as it comes back online from its closure due to the pandemic — could make the critical observations.
Additionally, the James Webb Space Telescope, slated to launch later this year, will be capable of directly imaging the host galaxy housing this quasar, peering through the not-yet-fully reionized intergalactic medium to see what no ground-based or space-based telescope presently operational can see.
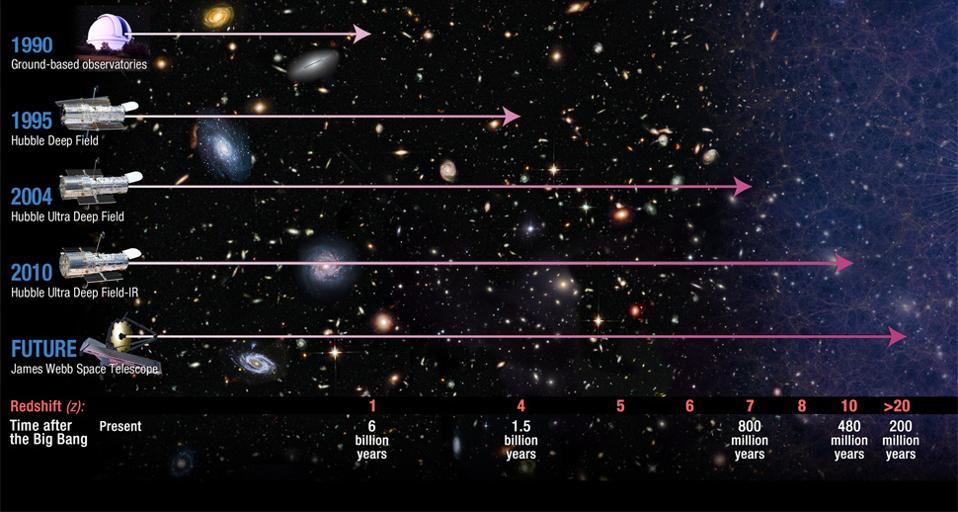
As we’re exploring more and more of the Universe, we’re able to look farther away in space, which … [+]
NASA / JWST and HST teams
It’s an absolutely remarkable thing that we’ve found a supermassive black hole at greater distances than ever before, in particular when we consider how rare these objects should be throughout the cosmos. But what’s truly puzzling is how this black hole got to be so big in such a short amount of time. Growing to 1.6 billion solar masses in a Universe that’s less than 700 million years old should only be possible, even if the black hole grows at the maximum rate allowed, if it starts off with about 10,000 solar masses: about a factor of 10 greater than realistic values admit.
Fortunately, we have near-future observations that will teach us much more about this object, including what its host galaxy is like and what the quasar outflows are doing. Over the coming years, we can expect to find even greater numbers of black holes in these far recesses of the Universe, as astronomers hope to learn how these objects actually form and evolve over time. For right now, we do not know how these black holes got so big so quickly in the early Universe, but the data doesn’t lie. These objects are out there, and it’s on us to figure out where they came from.